What is the Supercapacitor Structure Like?
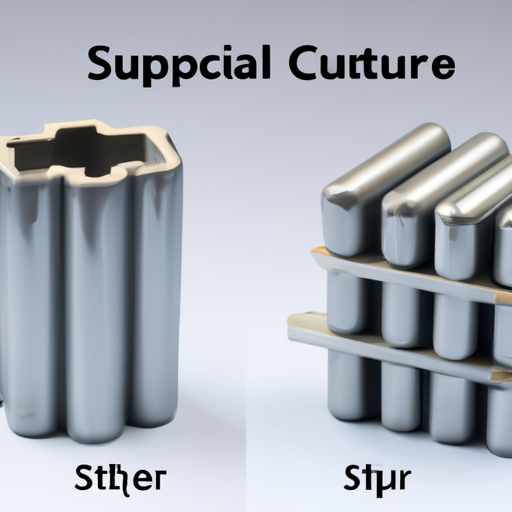
I. Introduction
In the realm of energy storage, supercapacitors have emerged as a pivotal technology, bridging the gap between traditional capacitors and batteries. Defined as electrochemical devices that store energy through electrostatic and electrochemical processes, supercapacitors are known for their ability to deliver rapid bursts of power and their long cycle life. As the demand for efficient energy storage solutions grows, understanding the structure and function of supercapacitors becomes increasingly important. This article will delve into the basic principles of supercapacitors, their components, types, manufacturing techniques, performance characteristics, applications, and future trends.
II. Basic Principles of Supercapacitors
Supercapacitors operate on two primary energy storage mechanisms: electrostatic double-layer capacitance and pseudocapacitance.
A. Energy Storage Mechanisms
1. **Electrostatic Double-Layer Capacitance**: This mechanism involves the formation of an electric double layer at the interface between the electrode and the electrolyte. When a voltage is applied, ions from the electrolyte accumulate at the electrode surface, creating a separation of charge that stores energy.
2. **Pseudocapacitance**: In addition to electrostatic storage, pseudocapacitance involves faradaic reactions, where charge transfer occurs at the electrode surface. This mechanism allows for higher energy storage compared to traditional capacitors, as it can store energy through reversible redox reactions.
B. Comparison with Traditional Capacitors and Batteries
Unlike traditional capacitors, which store energy purely through electrostatic means, supercapacitors can achieve higher energy densities, making them suitable for applications requiring quick bursts of power. In contrast to batteries, supercapacitors can charge and discharge much faster, but they typically have lower energy density. This unique combination of characteristics positions supercapacitors as a versatile energy storage solution.
III. Components of a Supercapacitor
The structure of a supercapacitor consists of three main components: electrodes, electrolytes, and separators.
A. Electrodes
1. **Materials Used**: The choice of electrode material is crucial for the performance of supercapacitors. Common materials include activated carbon, which offers a high surface area, graphene, known for its excellent conductivity, and metal oxides, which can enhance pseudocapacitance.
2. **Structure and Surface Area Considerations**: The surface area of the electrodes directly influences the capacitance. A higher surface area allows for more charge storage, making porous materials essential in electrode design.
B. Electrolyte
1. **Types of Electrolytes**: Supercapacitors can utilize various electrolytes, including aqueous solutions, organic solvents, and ionic liquids. Each type has its advantages and disadvantages in terms of conductivity, operating voltage, and temperature stability.
2. **Role of the Electrolyte in Performance**: The electrolyte facilitates ion transport between the electrodes, significantly impacting the supercapacitor's overall performance. The choice of electrolyte can affect the energy density, power density, and operational voltage range.
C. Separator
1. **Function and Materials Used**: The separator is a critical component that prevents direct contact between the electrodes, which could lead to short circuits. Common materials for separators include porous polymers and cellulose-based materials.
2. **Importance of Preventing Short Circuits**: A well-designed separator ensures that ions can pass through while maintaining electrical isolation between the electrodes, thus enhancing the safety and efficiency of the supercapacitor.
IV. Types of Supercapacitor Structures
Supercapacitors can be categorized into three main types based on their structure and operation.
A. Symmetric Supercapacitors
Symmetric supercapacitors use the same material for both electrodes, typically activated carbon. They are known for their simplicity and are widely used in applications where balanced energy and power density are required.
B. Asymmetric Supercapacitors
Asymmetric supercapacitors utilize different materials for the positive and negative electrodes, allowing for higher energy density. This design can combine the benefits of both capacitors and batteries, making them suitable for applications requiring higher energy storage.
C. Hybrid Supercapacitors
Hybrid supercapacitors combine features of supercapacitors and batteries, often using a battery-type material for one electrode and a supercapacitor material for the other. This structure allows for enhanced energy density while maintaining fast charge/discharge capabilities.
V. Manufacturing Techniques
The production of supercapacitors involves several key manufacturing techniques.
A. Methods for Electrode Fabrication
1. **Chemical Vapor Deposition (CVD)**: This technique is used to create thin films of materials like graphene, providing high-quality electrodes with excellent conductivity.
2. **Sol-Gel Process**: This method allows for the synthesis of metal oxides and other materials, enabling the creation of porous structures that enhance capacitance.
3. **Other Techniques**: Additional methods, such as electrospinning and 3D printing, are being explored to create novel electrode structures with tailored properties.
B. Assembly of Supercapacitors
1. **Layering and Stacking Methods**: The assembly process often involves layering the electrodes, separator, and electrolyte in a specific order to optimize performance.
2. **Encapsulation Techniques**: Proper encapsulation is essential to protect the supercapacitor from environmental factors and ensure long-term stability.
VI. Performance Characteristics
The performance of supercapacitors is characterized by several key metrics.
A. Capacitance and Energy Density
Capacitance is a measure of a supercapacitor's ability to store charge, while energy density indicates how much energy can be stored per unit volume or mass. Higher capacitance and energy density are desirable for efficient energy storage.
B. Power Density and Charge/Discharge Rates
Power density refers to the rate at which energy can be delivered, making it crucial for applications requiring quick bursts of power. Supercapacitors excel in this area, often achieving high charge and discharge rates.
C. Cycle Life and Stability
Supercapacitors are known for their long cycle life, often exceeding hundreds of thousands of charge/discharge cycles. Stability over time is essential for applications in various industries, ensuring reliability and performance.
VII. Applications of Supercapacitors
Supercapacitors find applications across a wide range of industries.
A. Consumer Electronics
In consumer electronics, supercapacitors are used to provide quick bursts of power for devices like cameras, smartphones, and wearables, enhancing performance and extending battery life.
B. Electric Vehicles
In electric vehicles, supercapacitors can complement batteries by providing additional power during acceleration and regenerative braking, improving overall efficiency.
C. Renewable Energy Systems
Supercapacitors play a vital role in renewable energy systems, such as solar and wind, by storing excess energy and providing it during peak demand periods.
D. Industrial Applications
In industrial settings, supercapacitors are used for energy storage in uninterruptible power supplies (UPS), grid stabilization, and various automation processes.
VIII. Future Trends and Innovations
The future of supercapacitors is promising, with several trends and innovations on the horizon.
A. Advances in Materials Science
Research into new materials, such as nanomaterials and composites, is expected to enhance the performance of supercapacitors, leading to higher energy densities and improved efficiency.
B. Integration with Other Energy Storage Technologies
The integration of supercapacitors with batteries and other energy storage technologies is likely to create hybrid systems that leverage the strengths of each technology, optimizing energy storage solutions.
C. Potential for Miniaturization and Flexibility
As technology advances, the potential for miniaturization and flexible supercapacitors opens up new possibilities for applications in wearable technology and portable devices.
IX. Conclusion
In summary, supercapacitors represent a significant advancement in energy storage technology, characterized by their unique structure and performance capabilities. Understanding the components, types, and manufacturing techniques of supercapacitors is essential for harnessing their potential in various applications. As research and innovation continue to drive the development of supercapacitors, their role in the future of energy storage solutions will undoubtedly expand, offering new opportunities for efficiency and sustainability in our increasingly energy-dependent world.
What is the Supercapacitor Structure Like?
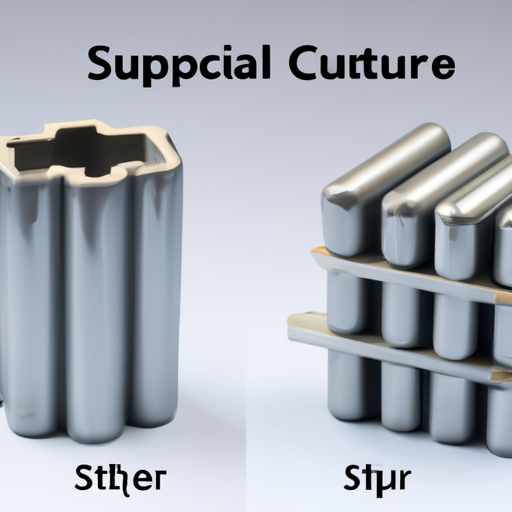
I. Introduction
In the realm of energy storage, supercapacitors have emerged as a pivotal technology, bridging the gap between traditional capacitors and batteries. Defined as electrochemical devices that store energy through electrostatic and electrochemical processes, supercapacitors are known for their ability to deliver rapid bursts of power and their long cycle life. As the demand for efficient energy storage solutions grows, understanding the structure and function of supercapacitors becomes increasingly important. This article will delve into the basic principles of supercapacitors, their components, types, manufacturing techniques, performance characteristics, applications, and future trends.
II. Basic Principles of Supercapacitors
Supercapacitors operate on two primary energy storage mechanisms: electrostatic double-layer capacitance and pseudocapacitance.
A. Energy Storage Mechanisms
1. **Electrostatic Double-Layer Capacitance**: This mechanism involves the formation of an electric double layer at the interface between the electrode and the electrolyte. When a voltage is applied, ions from the electrolyte accumulate at the electrode surface, creating a separation of charge that stores energy.
2. **Pseudocapacitance**: In addition to electrostatic storage, pseudocapacitance involves faradaic reactions, where charge transfer occurs at the electrode surface. This mechanism allows for higher energy storage compared to traditional capacitors, as it can store energy through reversible redox reactions.
B. Comparison with Traditional Capacitors and Batteries
Unlike traditional capacitors, which store energy purely through electrostatic means, supercapacitors can achieve higher energy densities, making them suitable for applications requiring quick bursts of power. In contrast to batteries, supercapacitors can charge and discharge much faster, but they typically have lower energy density. This unique combination of characteristics positions supercapacitors as a versatile energy storage solution.
III. Components of a Supercapacitor
The structure of a supercapacitor consists of three main components: electrodes, electrolytes, and separators.
A. Electrodes
1. **Materials Used**: The choice of electrode material is crucial for the performance of supercapacitors. Common materials include activated carbon, which offers a high surface area, graphene, known for its excellent conductivity, and metal oxides, which can enhance pseudocapacitance.
2. **Structure and Surface Area Considerations**: The surface area of the electrodes directly influences the capacitance. A higher surface area allows for more charge storage, making porous materials essential in electrode design.
B. Electrolyte
1. **Types of Electrolytes**: Supercapacitors can utilize various electrolytes, including aqueous solutions, organic solvents, and ionic liquids. Each type has its advantages and disadvantages in terms of conductivity, operating voltage, and temperature stability.
2. **Role of the Electrolyte in Performance**: The electrolyte facilitates ion transport between the electrodes, significantly impacting the supercapacitor's overall performance. The choice of electrolyte can affect the energy density, power density, and operational voltage range.
C. Separator
1. **Function and Materials Used**: The separator is a critical component that prevents direct contact between the electrodes, which could lead to short circuits. Common materials for separators include porous polymers and cellulose-based materials.
2. **Importance of Preventing Short Circuits**: A well-designed separator ensures that ions can pass through while maintaining electrical isolation between the electrodes, thus enhancing the safety and efficiency of the supercapacitor.
IV. Types of Supercapacitor Structures
Supercapacitors can be categorized into three main types based on their structure and operation.
A. Symmetric Supercapacitors
Symmetric supercapacitors use the same material for both electrodes, typically activated carbon. They are known for their simplicity and are widely used in applications where balanced energy and power density are required.
B. Asymmetric Supercapacitors
Asymmetric supercapacitors utilize different materials for the positive and negative electrodes, allowing for higher energy density. This design can combine the benefits of both capacitors and batteries, making them suitable for applications requiring higher energy storage.
C. Hybrid Supercapacitors
Hybrid supercapacitors combine features of supercapacitors and batteries, often using a battery-type material for one electrode and a supercapacitor material for the other. This structure allows for enhanced energy density while maintaining fast charge/discharge capabilities.
V. Manufacturing Techniques
The production of supercapacitors involves several key manufacturing techniques.
A. Methods for Electrode Fabrication
1. **Chemical Vapor Deposition (CVD)**: This technique is used to create thin films of materials like graphene, providing high-quality electrodes with excellent conductivity.
2. **Sol-Gel Process**: This method allows for the synthesis of metal oxides and other materials, enabling the creation of porous structures that enhance capacitance.
3. **Other Techniques**: Additional methods, such as electrospinning and 3D printing, are being explored to create novel electrode structures with tailored properties.
B. Assembly of Supercapacitors
1. **Layering and Stacking Methods**: The assembly process often involves layering the electrodes, separator, and electrolyte in a specific order to optimize performance.
2. **Encapsulation Techniques**: Proper encapsulation is essential to protect the supercapacitor from environmental factors and ensure long-term stability.
VI. Performance Characteristics
The performance of supercapacitors is characterized by several key metrics.
A. Capacitance and Energy Density
Capacitance is a measure of a supercapacitor's ability to store charge, while energy density indicates how much energy can be stored per unit volume or mass. Higher capacitance and energy density are desirable for efficient energy storage.
B. Power Density and Charge/Discharge Rates
Power density refers to the rate at which energy can be delivered, making it crucial for applications requiring quick bursts of power. Supercapacitors excel in this area, often achieving high charge and discharge rates.
C. Cycle Life and Stability
Supercapacitors are known for their long cycle life, often exceeding hundreds of thousands of charge/discharge cycles. Stability over time is essential for applications in various industries, ensuring reliability and performance.
VII. Applications of Supercapacitors
Supercapacitors find applications across a wide range of industries.
A. Consumer Electronics
In consumer electronics, supercapacitors are used to provide quick bursts of power for devices like cameras, smartphones, and wearables, enhancing performance and extending battery life.
B. Electric Vehicles
In electric vehicles, supercapacitors can complement batteries by providing additional power during acceleration and regenerative braking, improving overall efficiency.
C. Renewable Energy Systems
Supercapacitors play a vital role in renewable energy systems, such as solar and wind, by storing excess energy and providing it during peak demand periods.
D. Industrial Applications
In industrial settings, supercapacitors are used for energy storage in uninterruptible power supplies (UPS), grid stabilization, and various automation processes.
VIII. Future Trends and Innovations
The future of supercapacitors is promising, with several trends and innovations on the horizon.
A. Advances in Materials Science
Research into new materials, such as nanomaterials and composites, is expected to enhance the performance of supercapacitors, leading to higher energy densities and improved efficiency.
B. Integration with Other Energy Storage Technologies
The integration of supercapacitors with batteries and other energy storage technologies is likely to create hybrid systems that leverage the strengths of each technology, optimizing energy storage solutions.
C. Potential for Miniaturization and Flexibility
As technology advances, the potential for miniaturization and flexible supercapacitors opens up new possibilities for applications in wearable technology and portable devices.
IX. Conclusion
In summary, supercapacitors represent a significant advancement in energy storage technology, characterized by their unique structure and performance capabilities. Understanding the components, types, and manufacturing techniques of supercapacitors is essential for harnessing their potential in various applications. As research and innovation continue to drive the development of supercapacitors, their role in the future of energy storage solutions will undoubtedly expand, offering new opportunities for efficiency and sustainability in our increasingly energy-dependent world.